Mapping the carbon capture landscape
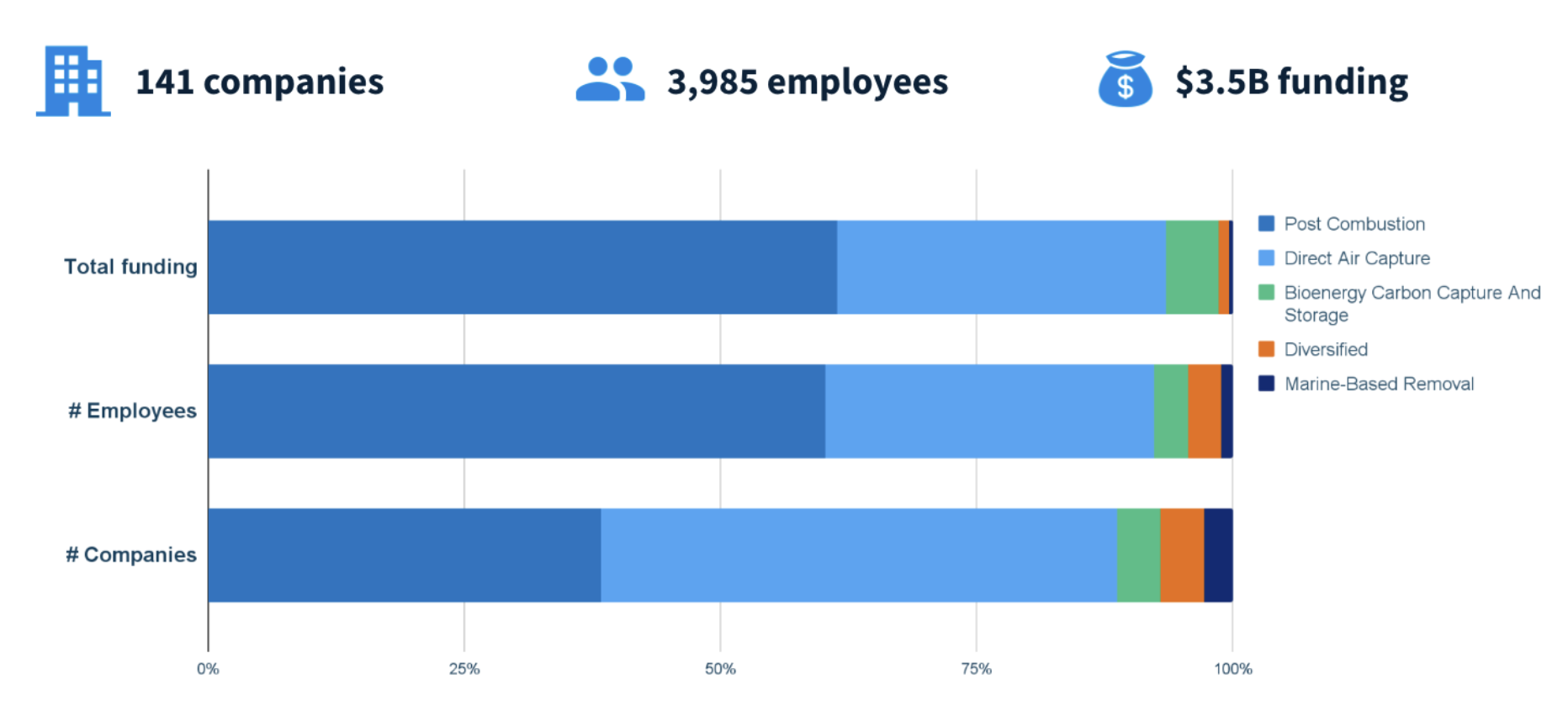
We analyzed the carbon capture market and assessed the most promising technologies. Read further to learn about the latest developments and controversies.
The carbon capture landscape encompasses a suite of technologies designed to capture and remove carbon dioxide (CO2) from diverse sources. Along with a remarkable surge in investment, reaching an unprecedented milestone of $6.4 billion in 2022, this field has witnessed a growing wave of criticism and skepticism. Behind this spotlight lies the pivotal role that carbon capture technologies could play in achieving global energy and climate goals. Most net-zero scenarios presented by the world’s leading organizations, including the Intergovernmental Panel on Climate Change (IPCC), the International Energy Agency (IEA), and Bloomberg New Energy Finance (BNEF), depend critically on carbon capture expanding to 6-8 Gt of CO₂/year to reach net-zero emissions by 2050.
The topic of carbon capture has sparked polarizing opinions over the years. While proponents praise it as essential to limit warming to 1.5 degrees C and especially vital for hard-to-abate industries, skeptics condemn it as a greenwash to prolong the use of fossil fuel assets. In the next paragraph, we delve into the merits of these two contrasting positions, shedding light on the true potential and inherent limitations of carbon capture technologies.
In the fight against climate change, carbon capture emerges as a powerful tool, operating through three key channels:
From an environmental perspective, carbon capture's potential applications in gas processing and power generation are less favorable. Gas processing has been the main carbon capture application, driven by the necessity to eliminate CO2 from the extracted raw gas. While the application has historical roots, its usage has primarily been pragmatic rather than environmentally motivated. Power generation is a newer use case of CCS to decarbonize the power sector. However, the adoption cost for this application remains comparably high, with carbon capture competing with renewables in the power sector as a climate change mitigation solution.
The leading criticism against carbon capture is well summarized by a recent article by The Guardian. The journalist George Monbiot states that “the sole purpose of CCS is to justify the granting of more oil and gas licenses, on the grounds that one day someone might be able to capture and bury the CO2 they produce”, further criticizing “most of the very few projects” for having been “abject failures”. The skepticism surrounding carbon capture technology primarily centers around two key concerns: its scope for sustainability purposes and the efficacy of the technology itself.
The allegation that carbon capture might merely serve as a form of "greenwashing" to extend the lifespan of fossil fuels stems from the fact that the initial and predominant commercial applications of carbon capture technologies were directed toward enhanced oil recovery and natural gas processing. These applications were historically driven by economic motives rather than environmental ones, resulting in commercial advancements limited to the economic realm. It wasn't until the 1990s that the narrative around utilizing CO2 began to shift from purely economic considerations to an environmental perspective, positioning carbon capture as a potential solution to address climate change.
The concern that “based on historical trajectories, much of this captured carbon will be used for enhanced oil recovery” is a valuable cautionary note that should guide the technology's evolution. Nevertheless, this apprehension shouldn't impede the ongoing progress in its development. An illustrative example is the Sleipner Project in Norway, which stands out as a remarkable success story in this arena. It notably became the pioneer in capturing CO2 without using it for enhanced oil recovery. Instead of venting the gas, the facility injects CO2 in a dedicated geological structure, consistently reaching its target capacity of 0.9 million tons (Mt)/year with no evidence of leakage.
The Sleipner project isn’t just an example of a well-functioning CCS facility. It shows how politics and economics can work together to tackle climate change. By introducing a CO2 tax costing operators $49 per tonne of CO2 in 1991, Norway made carbon capture both environmentally sound and financially sustainable. With the cost of injecting CO2 around $17 per tonne and a total investment of $100 million to build the facility, operators could break even in just a few years.
The success of projects like Sleipner and the later Snøhvit, another Norwegian project commissioned in 2007, can be attributed to the presence of a stringent regulatory environment. These instances demonstrate that carbon capture can be environmentally friendly, provided it is incentivized by a strong and well-crafted regulatory framework.
While the initial critique focuses on technology usage rather than performance, carbon capture's effectiveness and feasibility have also encountered robust scrutiny.
A recent study by the Institute for Energy Economics and Financial Analysis (IEEFA) examined 13 global carbon capture projects, classifying them based on their application and sharing lessons from their technical and economic performance. The summary of findings presented by the authors paints a bleak picture: seven projects underperformed, one was questionable, and just two gas processing projects in Norway succeeded. A closer examination of the report reveals a less pessimistic outlook.
The study analyzed five flagship gas processing projects, with two succeeding due to robust regulation. Yet, not all results were dismal; the largest project, operating for 35 years, recently improved to nearly meet the capture target (about 75% of total CO2 emissions). A weak performer and a suspended project provide valuable insights into areas requiring further development. Notably, refining regulations and advancing our understanding of geo-mechanical responses to CO2 injections are crucial to avert failures and leaks.
In the power sector, three projects were assessed, with two failing and one mothballed. Reasons vary from missing carbon sequestration targets to technical issues and oil price fluctuations. Carbon capture faces more complexities here due to higher costs and lower CO2 concentrations in coal plants. Economic viability often leads to selling CO2 for oil refining, thwarting environmental goals and triggering critics.
Five projects in industrial applications, including hydrogen, chemicals, ethanol, fertilizers, and hard-to-abate industries, were also studied. Amidst the report's negativity, positives emerge. The hydrogen project meets expectations, and even accounting for additional emissions from capture operations, net avoided CO2 was 4.22MT in five years. Similar results surfaced across subsectors. The authors fail to assess the only operational project in hard-to-abate industries due to limited data availability. However, they conclude that carbon capture in hard-to-abate industries holds the greatest promise since CO2 is an inevitable by-product of chemical reactions in cement production.
Stepping back, across their entire investigation, the authors consistently point out carbon capture's shortcomings in comparison to renewable energy in terms of environmental impact. While this assessment holds merit, given the pressing urgency of our current situation, a solely renewable energy approach falls short. Thus, a combined strategy is essential to effectively scale up a decarbonized energy supply. Guided by insights from previous projects, the best course of action involves:
Having discussed historical data and operational projects in the preceding chapter, our focus now shifts to gaining a deeper understanding of the carbon capture industry through our expertise: scrutinizing ongoing and forthcoming technological advancements by exploring startups and innovation initiatives.
Venture IQ has compiled an extensive dataset of 153 companies active in carbon capture. To segment carbon capture technologies effectively, we've employed a categorization based on the point of capture.
Since companies can be active in more segments, we introduce a “diversified” category in the following sections of this report to distinguish companies that utilize two or more of these processes.
In recent years, the carbon capture sector has witnessed a remarkable surge in the establishment of startups, marking a significant milestone after decades of modest development. This surge can be attributed to the implementation of favorable policies and incentives, particularly in North America and Europe, which have played a crucial role in fostering the growth of this industry. For a complete overview of policy developments, we recommend consulting the IEA Policies database.
Despite the popularity of direct air capture (DAC) solutions, particularly on the startup scene, solutions capturing carbon after combustion are leading in terms of funding and workforce size.
Post-combustion solutions have emerged as a favorable choice for deploying carbon capture due to their retrofitting capabilities, eliminating the need for constructing entirely new facilities. This advantage makes them a preferred option over DAC, which, despite offering flexibility in terms of location, tends to consume higher energy and thus presents less enticing investment opportunities. Additionally, post-combustion companies stand out as the most mature among the various options available in the market.
Post-combustion is a dominant force in the industry. The combination of its market dominance, maturity, and positive growth indicators positions post-combustion as a key segment shaping the industry's future landscape.
In addition to this established technology, our spotlight turns to the swiftly emerging marine-based removal technique. This method captures CO2 from marine environments, particularly oceans, benefitting from their notably higher volumetric CO2 concentration—approximately 150 times that of the air. This inherent efficiency reduces the need for extensive water pumping, setting it apart from the air-vacuuming process of direct air capture.
Bioenergy carbon capture also commands attention as a remarkable approach, being the only carbon dioxide removal technique that can also provide energy. While the technology is attracting growing funding and targeted BECCS programs are emerging in the US and Europe, adoption remains limited, and technological advancement is in the early stages.
Regardless of the chosen capture technology, a discernible pattern surfaces in our analysis. While diverse players prioritize different capture points, the common denominator among early-stage startups is their shared commitment to increasing energy efficiency. If you’re curious to learn more, we've featured a compilation of noteworthy carbon capture startups in our separate blog article.
Venture IQ is a consultancy firm with expertise in scouting technology companies. With Catalist, our dedicated software platform, we can help our clients to uncover hidden gems and save time on getting a complete overview of companies in any market. Over the past 7 years, Venture IQ has been able to help 100+ corporates, VC, and PE funds with their challenges in finding exciting investment opportunities and insights on new technological developments.
Want to learn more about how Venture IQ can help with technology scouting? Feel free to book a meeting or read more about our market mapping services.